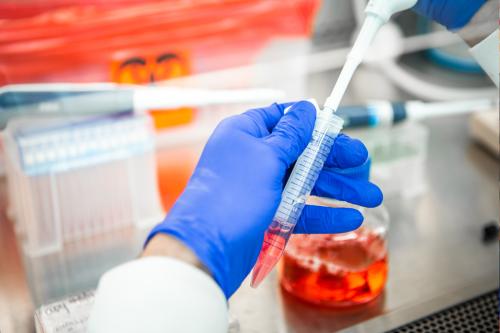
BSCRC awards grants to 12 game-changing stem cell research and technology projects
Each year, the Eli and Edythe Broad Center of Regenerative Medicine and Stem Cell Research at UCLA funds a select number of promising research projects that could accelerate the development and application of life-changing stem cell therapies.
This year, the center has granted $1.5 million in awards to 12 projects tackling critical challenges in areas ranging from neurological diseases to genetic limb malformations.
The Innovation Awards support scientists pursuing their most bold and ambitious research ideas that seed the ground for new discoveries that could lead to therapies for patients who need them most. Launched this year, the Transformative Technology Development Awards provide funding for center members seeking to develop innovative technologies with high potential to advance stem cell research.
The awards have been generously funded in part by the Alfred E. Mann Charities, The Gillian S. Fuller Foundation, The Rose Hills Foundation Innovator Grant Program and Carol Doumani.
Innovation Awards
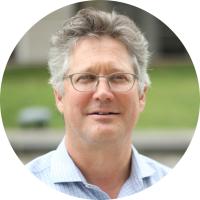
Principal investigator: Douglas Black, Ph.D.
Project: “Spliced isoforms of DPF2 affecting chromatin and gene expression programs of ESC pluripotency and skeletal muscle development”
DPF2 is a protein that rearranges the structure of chromatin, a mixture of DNA and proteins in which genes are packaged. The Black lab recently discovered that changes to the mRNA that encodes DPF2 affect the regulatory programs involved in embryonic stem cell maintenance and the development of neurons. This project investigates how similar changes in DPF2 mRNA affect the biology of muscle cells to uncover the protein's impact on muscle development and ultimately shed light on its contributions to musculoskeletal diseases such as Coffin-Siris syndrome.
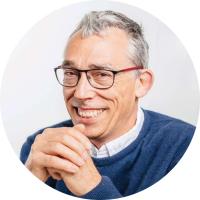
Principal investigator: Jonathan Flint, M.D.
Co-investigators: Kathrin Plath, Ph.D.; Bennett Novitch, Ph.D.
Project: “Generation of hPSC lines to report the state of neuronal maturation and investigate developmental timing mechanisms”
This project aims to address a key question in neurobiology: How do neurons know when to mature? Mouse neurons take three weeks to mature while human neurons take years, yet the neurons in both species look the same. So, what determines the rate of maturation? To address this question, the research team will add fluorescently labeled proteins that appear at different points on the neuronal development timeline. This will allow the scientists to observe the maturation process as human pluripotent stem cells develop into neurons and identify the genes that control neuronal maturation. This new tracking system has the potential to not only uncover a fundamental process involved in brain growth, but also provide key insights into the causes of mental disorders.
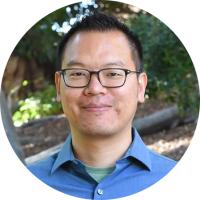
Principal investigator: Jimmy Hu, Ph.D.
Co-investigator: Neil Lin, Ph.D.
Project: “Regulation of dental epithelial stem cells by cell geometries and jamming transition”
Human teeth have limited regenerative potential and current restorative treatments for missing or damaged teeth like dental implants are imperfect or temporary. Using mouse teeth as a model, scientists are integrating recent breakthroughs in imaging, genomics and AI technology to reveal how cell shapes and motility influence gene expression. This knowledge will directly inform new cell geometry-based strategies to guide the fabrication of natural teeth using dental stem cells.
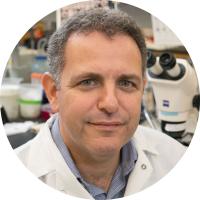
Principal investigator: Bennett Novitch, Ph.D.
Co-investigator: Neil Lin, Ph.D.
Project: “Modeling the impact of oxygenation on human brain development and functional maturation using iPSC-derived brain organoids”
Brain organoids are offering researchers an intimate look at the complexities of human brain development and disease. However, there are doubts about their accuracy as models due to their lack of vascularization, a deficiency that could lead to irregular cell development and hindered maturation. This project seeks to overcome these limitations by establishing a reliable method to increase nutrient exchange and oxygenation in organoids in the absence of vascularization. Initial studies have shown that these modifications led to noticeable improvements in the brain organoids’ overall health and growth, suggesting that such changes could maximize their potential in accurately modeling neurological disorders.
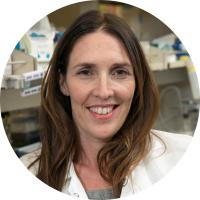
Principal investigator: April Pyle, Ph.D.
Project: “Human limb formation from pluripotent stem cells”
Limb defects are one of the most common birth defects, occurring in approximately 1 out of every 200 live births. Researchers are using human pluripotent stem cells to develop a 3D model that will provide the instructions to guide the formation of the limb bud (the earliest limb structures in human development), its outgrowth and its subsequent digit formation. This work could result in the first human model of limb malformations. It could also lead to a better understanding of the mutations that lead to these defects, a key step towards the development of gene correction strategies that can fully reverse the causes of limb malformations in humans.
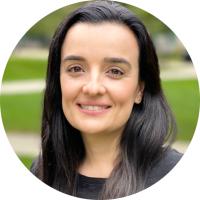
Principal investigator: Débora Sobreira, Ph.D.
Project: “Establishing a human iPSC-derived model for visceral white adipocytes”
Scientists are developing a human induced pluripotent stem cell-derived model that’s specifically tailored to study visceral white adipocytes, a pivotal but understudied cell type in metabolic health and disease. By using iPSCs sourced from diverse origins, this model can help shed light on the genetic and biological mechanisms behind metabolic disorders such as obesity and Type 2 diabetes. The system could also help researchers study drug mechanisms, assess therapy effectiveness and toxicity, and develop personalized treatments for metabolic diseases.
Transformative Technology Development Awards
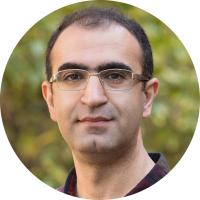
Principal investigator: Amjad Askary, Ph.D.
Co-investigator: Amander Clark, Ph.D.
Project: “Recording and reconstruction of signaling activity in human pluripotent stem cells”
Scientists are developing a new molecular recording approach that provides access to the history of a single cell’s signaling activity based on endpoint measurements. By using this method in human pluripotent stem cells to record two main developmental signaling pathways, WNT and BMP, researchers will gain a deeper understanding of how these pathways influence cell fate decisions across a broad range of stem cell-based models. Further applying this approach in a stem cell-derived embryo model will enable researchers to study otherwise inaccessible stages of human development that are critically important in early pregnancy loss and infertility.
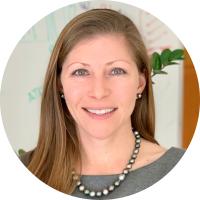
Principal investigator: Heather Christofk, Ph.D.
Project: “CYTO-Tag for measuring tissue stem cell metabolism in vivo”
Researchers have developed a novel technology that enables scientists to study the unique metabolism of individual cell types within the body. Now, this team of investigators is applying this new technology to determine the metabolic properties of two types of tissue stem cells: stem cells in the intestine and the muscle. By analyzing how intestinal stem cell metabolism changes with fasting, diet and pregnancy, as well as how muscle stem cell metabolism changes with injury, exercise and aging, researchers can better understand metabolism’s influence on the body.
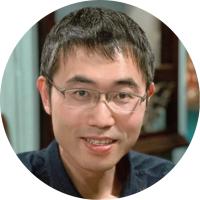
Principal investigator: Liang Gao, Ph.D.
Project: “High-throughput label-free metabolic imaging of organoids”
Organoids are revolutionizing the study and treatment of diseases, but researchers are finding it increasingly challenging to image them effectively as these 3D models become more complex. This project aims to develop a new imaging technique that will allow scientists to capture detailed 3D images of organoids by transforming them into lines, which can then be analyzed using standard high-speed cameras.
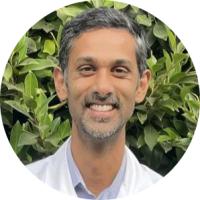
Principal investigator: Ranmal Samarasinghe, M.D., Ph.D.
Project: “A modular, cost-efficient and integrated platform for electrophysiological network analysis of brain organoids”
Almost every neurological disease is caused by dysfunction in a complex network of brain cells that communicate with each other through electrical impulses, so measuring the electrical network activities both in healthy brains and brains affected by neurological disease is crucial to uncovering disease pathways and identifying novel therapies. Brain organoids are often generated from the stem cells derived from patients with specific neurological diseases and are capable of producing in a dish some of the complex brain network activities that occur in human patients. However, the technologies required to measure these organoid brain network activities are expensive and technically challenging to use. This project aims to develop a single, cost-efficient and modular platform that will make these technologies much more accessible to all stem cell and organoid researchers.
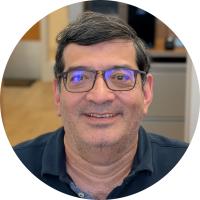
Principal investigator: James Wohlschlegel, Ph.D.
Co-investigators: Kathrin Plath, Ph.D.; Dino Di Carlo, Ph.D.
Project: “OMNI SEC-seq: Simultaneous analysis of the whole secretome and transcriptome of individual cells”
A bottleneck to unlocking the full therapeutic potential of stem cells to promote tissue regeneration is rooted in the heterogeneity of cell populations. While samples of cells might appear identical at first glance, they’re typically made up of sub-populations of cells that have diverse functional properties that lead to inconsistent clinical outcomes when used in therapeutic settings. A team of investigators is tackling this challenge by developing new methods for characterizing cells at the individual level instead of in mixtures. This will enable researchers to isolate single cells that are optimized for making a specific molecule of interest and then harnessing those capabilities in a wide range of clinical settings.
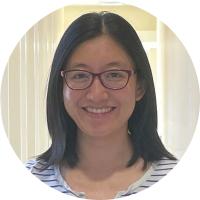
Principal investigator: Ye Zhang, Ph.D.
Co-investigator: James Wohlschlegel, Ph.D.
Project: “Developing novel tools to map the extracellular matrix of the stem cell niche”
Just like seeds need the right soil to grow well, stem cells need the right environment to stay healthy. However, current efforts to develop stem cell therapies mostly focus on making the stem cells themselves stronger, without much attention given to improving the environment into which they’ll be transplanted. This is because the tissue surrounding stem cells is complex and almost impossible to study with current technologies. The Zhang and Wohlschlegel labs seek to develop new tools that will enable scientists to identify the components of stem cells’ tissue environments and apply these insights to recreate the supportive elements needed for stem cells to thrive after transplantation. Such an advance could significantly improve stem cell survival after transplantation and potentially transform the treatment of a range of diseases that involve cell loss, such as stroke, Alzheimer's disease and heart failure.